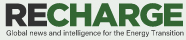
SPECIAL REPORT
Why shipping pure hydrogen around the world might already be dead in the
water
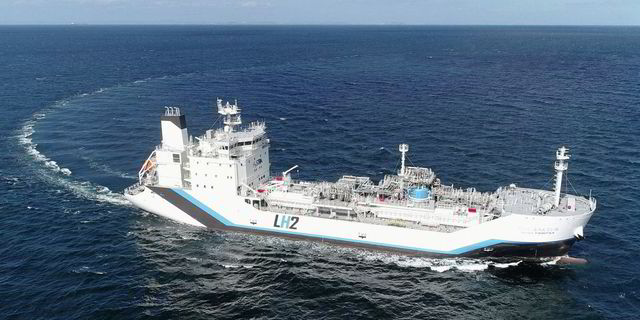
Physics and cost mean that ammonia is a far
more economic option for long-distance seaborne transportation, writes
Leigh Collins
The world’s first shipment of liquid
hydrogen — which left southern Australia on Monday on its way to Japan
as part of a $350m international project — may have made headlines
around the globe, but it might also prove to be a colossal waste of
money.
Putting aside the fact that the
Hydrogen Energy Supply Chain (HESC) project is ruthlessly
dirty — sourcing its H2 from unabated brown coal and using
polluting diesel to power the
Suiso Frontier vessel on its 20-day journey — the
notion that any commercial operator would choose to export pure
hydrogen by ship is looking increasingly unlikely.
This is not because of greenhouse gas
emissions, or the lack of vessels or suitable port facilities, but
purely on the grounds of physics and cost.
According to the International Renewable
Energy Agency, German think-tank Agora Energiewende and energy analyst
Wood Mackenzie, it would make economic sense to ship ammonia (NH3),
a hydrogen derivative, rather than pure H2.
On the surface, this might seem odd.
After all, hydrogen is the lightest and most energy-dense substance
(by weight) in the universe. So why would you want to make something
else out of the H2 and transport that instead?
As Noel Tomnay, WoodMac’s global head of
hydrogen consulting, wrote in
a recent article published by Recharge, there are
three reasons why ammonia is preferable to pure H2 for
long-distance exports — “its energy density; its proven synthesis
technology and existing supply chains; and its potential to drive
decarbonisation in its own right”.
Energy density
The truth is that hydrogen's
unsurpassable energy density by weight is irrelevant. When being
transported in giant metal tanks, what really matters is its energy
density by volume.
“Hydrogen transport by ship is
technically possible for larger distances where pipelines are not an
option. Because of its low energy density by volume, gaseous hydrogen
is best converted into a more energy-dense liquid before being loaded
onto a ship,” says Irena’s recent report,
Geopolitics of the Energy Transformation: The Hydrogen Factor.
“There are several vectors for hydrogen transport via ship, but
ammonia is the most promising.”
At normal atmospheric pressure, hydrogen
contains just 3kWh of energy per cubic metre, so it either has to be
compressed or liquefied to increase its energy density — to 1,411kWh/m3
(at a pressure of 700 bar), or 2,350kWh/m3 when
super-cooled to a liquid at a balmy minus 253°C.
The volumetric energy density of ammonia is 59% higher — at
3,730kWh/m3 when stored in its standard liquid form at
minus 33.3°C.
So, assuming same-sized vessels, it would theoretically take more
than three shipments of liquid hydrogen (LH2) to transport
the same amount of energy as two shipments of liquid ammonia (LNH3).
Production costs/trade
But isn’t it easier and cheaper to stick
to hydrogen, rather than using it to produce ammonia? After all, the
Haber-Bosch process, which combines nitrogen from the air with H2
to form NH3, is notoriously energy-intensive.
Well, according to the EU-funded hydrogen
liquefaction technology project,
IDEALHY, state-of-the-art technology today requires 12kWh
per kilo of liquid hydrogen (IDEALHY wants to reduce that to about
6kWh/kg).
And the traditional Haber-Bosch process,
which is usually powered by fossil fuels, requires 9-11kWh/kg,
according to multiple sources. However, powering Haber-Bosch via
renewable electricity could cut this to 6.41kWh/kg, say
researchers at the UK’s Cranfield University.
While that might sound like a win for
ammonia, LH2 actually contains a lot more energy per
kilogram than ammonia — 33.6kWh/kg versus 5.2kWh/kg. So while
conversion to LH2 requires the equivalent of 36% of the
energy that the hydrogen contains, producing ammonia requires more
energy than that held by the produced NH3.
So why on earth would it make more sense
to ship ammonia, rather than LH2?
First of all, we need to look at costs.
According to a 2019
report by the US Department of Energy, a new H2
liquefaction plant with a capacity of 27 tonnes per day would cost
$150m, a capital contribution of roughly $1.40 per kilo. Levelised
running costs would add up to a further $2.75/kg — and that’s not
including the price of producing the H2 in the first place.
According to Bunro Shiowaza, a senior
associate at the Sumitomo Chemical Company in Japan,
writing in the Japanese-language International Environment and
Economy Institute Journal, producing zero-carbon ammonia from
green hydrogen (priced at $3/kg) would cost $480 per tonne, or
$0.48/kg (note: ammonia is only 17.65% hydrogen by mass).
LH2 based on green hydrogen at
the same price would cost $7.15/kg — almost 15 times higher than green
NH3.
According to Recharge calculations
(see panel below), this means that a 160,000m3 cargo
(a standard LNG vessel size) of liquid hydrogen would cost about $200
per MWh to produce, in terms of the energy it contains, compared to
just under $88/MWh of liquid ammonia.
There are other advantages for ammonia,
not least because NH3 is already being transported
commercially on ships.
According to Tomnay, the seaborne trade
in ammonia amounts to about 20 million tonnes of liquid ammonia each
year.
By contrast, the commercial operation of
high-capacity LH2 ships is not targeted until the 2030s, at
least, not by HESC, according to
its website.
Tomnay adds that a world-scale ammonia
plant produces about two million tonnes per year, but the largest LH2
plants being proposed are in the range of 15,000-30,000 tonnes
annually, so scaling up the latter would be much harder.
Boil-off and shipping costs
Another significant reason to transport
ammonia rather than liquid hydrogen, is their boiling points — ie, the
temperature at which the liquid reverts to a gas.
Because LH2 needs to be stored
at a far colder temperature than ammonia (minus 253°C vs minus 33°C),
it is far more difficult to maintain that temperature over long
voyages — especially when vessels rely on insulation to keep these
liquids cold, rather than on-board refrigeration, such as the Suiso
Frontier.
Inevitably, a proportion of these
sub-zero liquids will warm up enough to turn into gas — what is known
in the industry as “boil-off”.
According to figures from a
November 2020 study by academics at the Hamad Bin Khalifa
University in Qatar, published in the journal Energy Reports,
transporting 160,000m3 of LH2 from Qatar to
Japan would result in an annual boil-off rate of 13.77%. This means
that 13.77% of its cargo weight would be lost over the course of a
year (24 voyages).
By contrast, a same-sized vessel
transporting 160,000m3 of liquid ammonia on the same route
would lose only 0.325% of its cargo weight to boil-off. (This low rate
could be due to the fact that existing ammonia carriers are often
fully refrigerated to keep the NH3 in its liquid state —
something that isn't possible with far-colder LH2.)
Using the aforementioned liquid-hydrogen
production price of $7.15/kg, that would translate as a $270.5m loss
from boil-off every year. By contrast, the annual losses from LNH3
boil-off along the same route, based on the production price of
$0.48/kg, would be just $4.1m.
It is important to point out, however,
that the LH2 figures would be redundant if the carrier
vessels had on-board reliquefaction systems, which captured the
boil-off, cooled it back to a liquid and pumped it back into the
storage tank.
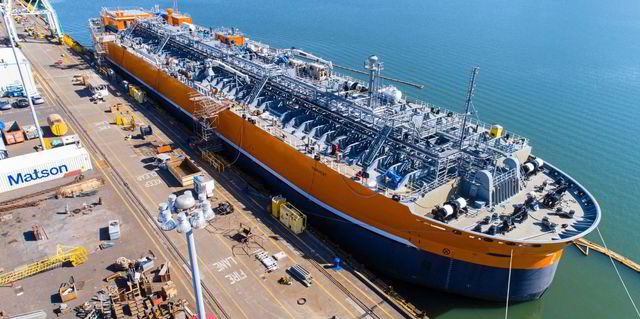
A fully refrigerated liquid-ammonia
carrier built by US shipbuilder Vigor Industrial. Photo: Vigor
Industrial
Such systems — which would add
considerable capital costs — are available for carriers of liquefied
natural gas (LNG, a liquid at minus 162°C). However, they are rare as
LNG boil-off is usually used to fuel the engines of the ship itself —
a possibility for future LH2- and ammonia-powered vessels.
According to the Qatari study, the
capital cost of a vessel (without reliquefaction facilities) carrying
160,000m3 of LH2 would be significantly higher
than one holding the same volume of LNH3 — $216m vs $162m —
although those figures are attributed by authors Mohammed Al-Breiki
and Yusuf Bicer to an earlier study from 2006.
While the paper points out that bunker
fuel costs would be a lot more for the ammonia vessel due to the
heavier weight of its cargo, when factoring in maintenance and other
expenses, the total ship operating costs for the year would only be
25.9% higher — $24.3m compared to $19.3m. No calculations are made for
future vessels powered by their own cargo.
Taking all these elements into account,
it is clear that ammonia would be far less expensive to transport by
sea than liquid hydrogen.
Agora calculates it would be cheaper to
produce green hydrogen in the EU than to import renewable H2
by ship from places such as Chile and Australia, where high solar
irradiation and strong winds means hydrogen can be produced extremely
cheaply.
However, as the think-tank explains in
its recent report, 12 Insights on Hydrogen, the opposite would
be true for hydrogen derivatives such as ammonia, methanol or
synthetic fuel.
“When the end-use molecule is hydrogen,
shipping from faraway lands such as Chile or Australia works out to be
more expensive than if the hydrogen was produced locally in Germany,
even with average renewables,” the study says.
“However, instead of cracking [ie,
converting] shipped ammonia back to hydrogen, using ammonia directly
as a fuel could be cheaper than the local production of hydrogen, even
in 2050.”
Use ammonia, not H2
You might be asking yourself at this
point, “We'll still need lots of pure hydrogen to decarbonise industry
and heavy transport, so what about the cost of converting the ammonia
back to hydrogen?” To which Recharge’s answer is this: “Why
would you want to?”
The global market for ammonia is
enormous, at
about 176 million tonnes per year, according to the UK’s
Royal Society. And as the IEA pointed out in its 2019
The Future of Hydrogen report, an estimated 31.5
million tonnes of hydrogen — roughly 42% of the annual global
production, almost all of which is derived from unabated fossil fuels
— was used to produce ammonia in 2018.
As the Royal Society policy briefing note
points out, ammonia production currently results in about 500 million
tonnes of CO2 being released into the atmosphere each year,
about 1.8% of annual global carbon emissions.
Replacing that dirty ammonia — most of
which supports food production — with green NH3 will be
vital if the planet is to reach net-zero emissions by 2050.
And, assuming an electrolyser efficiency
of 50kWh/kgH2, the green hydrogen required to do that would
need 1,575 terawatt hours of green energy — equivalent to 20% of
today’s annual global renewables production (8,300TWh, according to
the IEA’s
Global Energy Review 2021).
On top of that, more renewable energy
would be needed to power the Haber-Bosch process.
Developers moving to ammonia
Export-focused green-hydrogen project
developers have begun to take notice of the advantages of shipping
ammonia.
According to Wood Mackenzie, the majority
of export-oriented low-carbon (blue and green) hydrogen projects plan
to ship ammonia, rather than pure H2.
“More than 85% of the proposed capacity
integrates ammonia and hydrogen to some degree, with ammonia intended
for export markets and the remainder, hydrogen, largely aimed at
domestic markets,” wrote Tomnay.
He explains that ammonia could become the
marine fuel of choice, and is also being planned for use in power
generation, particularly in Japan, South Korea and Germany, so demand
for NH3 could well grow substantially in the coming years.
Tomnay agrees that it does not make sense
to convert ammonia back into hydrogen. “While ammonia is a potential
carrier of hydrogen that can be unlocked by cracking or a reversal of
the synthesis reaction, progressing this route at a large scale faces
technical and commercial challenges,” he wrote.
But don’t we still need to trade pure
hydrogen?
Yes, the world will still need a lot of
pure hydrogen, probably to decarbonise heavy industry and heavy
transport, rather than for use cases such as heating and cars, where
electric options will be far cheaper.
And countries such as Germany, Japan and
South Korea — as well as the EU — believe that they will not be able
to produce enough clean hydrogen locally to meet demand. So
international trade in H2 is very much still needed.
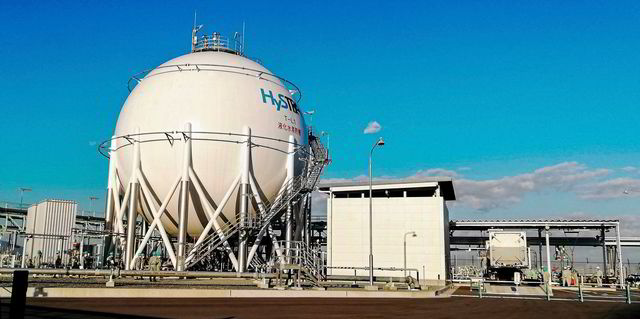
The liquid hydrogen storage tank in
Kobe, Japan, which is awaiting the shipment of LH2 aboard the Suiso
Frontier. Photo: AFP/Getty
Agora’s recent hydrogen report says that
the markets for pure H2 will become regional, as
transporting the element by long-distance pipeline will be roughly
half the price of shipping it to Europe.
“In practice, then, opportunities for
ship-based hydrogen trade will be limited to instances where pipelines
are not ready or unfeasible due to, say, public opposition or distance
(as in Japan) or politics,” it says.
While European nations could
theoretically receive its H2 supply by pipes from the
Middle East or North Africa, more isolated countries such as South
Korea and Japan, will not be able to — even though they are both
planning to import huge amounts of hydrogen in their respective bids
to reach net-zero emissions.
For these nations, something more radical
might be needed.
Relocating industry
In its hydrogen geopolitics report, Irena
makes the point that it would actually be cheaper, in some cases, to
move industrial facilities to sun- or wind-rich parts of the world
where green hydrogen would be very cheap to produce and then to
transport the final product made using that H2, rather than
transporting the hydrogen itself.
“The cost of transporting renewable
energy, whether in the form of electricity or hydrogen, remains
relatively high,” it says. “The cheapest way to transport energy is in
materials and products. Thus, renewable potentials create a
significant competitive advantage for regions with surplus renewable
resources to become sites of green industrialisation.
“Relocating industry makes sense where
the energy cost reduction exceeds the additional shipping cost.
Relocation may benefit commodities such as aluminium, ammonia, iron,
jet fuel and methanol.”
So even exporting ammonia around the
world may not be the lowest-cost option for some companies.
“Some energy-intensive industries may
relocate to countries with low-cost renewable surpluses, exporting
commodities or semi-finished products (direct reduced iron, etc.) for
finishing in other countries,” the report adds.
The report does add the caveat that
choosing industrial facility locations depends on more than just cheap
energy.
“Existing industrial clusters and
agglomerations are likely to be resistant to change and exhibit path
dependency. Most low-carbon steel plants in Europe, for example, are
located within existing industrial clusters. Moreover, countries will
want to retain their industrial base while looking for ways to
decarbonise polluting industries.”
But it adds: “Setting up new production
facilities in renewables-rich countries does not necessarily imply the
closure of plants elsewhere. On the contrary, in many industries,
there is scope for growth. By 2050, around 200 million tonnes per year
of expected global steel demand cannot be met by retrofitting existing
production sites. New opportunities will exist to set up additional
clean production facilities in countries with iron ore and cheap
renewables.”
And it cites previous examples of such
industrial relocations based on access to cheap energy.
“Following the oil crises in the 1970s,
Japan phased out aluminium smelters and switched to imports. Aluminium
smelters are typically situated close to hydropower dams with large
amounts of low-cost electricity, in places as diverse as Canada,
Iceland, Mozambique, Norway, Russia, Suriname, Tajikistan and
Venezuela. Ammonia plants have been located close to sources of
low-cost natural gas, in Norway, the Middle East and Russia, for
example.”
So perhaps the high cost of shipping pure
hydrogen will lead to a new era of industrial development in sparsely
populated coastal desert regions, where winds and sunshine are both
strong — places such as Chile, Australia, Namibia, Saudi Arabia and
Oman, all of which are planning gigawatt-scale green hydrogen
projects, coupled with ammonia production.
How Recharge calculated
the cost of shipfuls of ammonia and liquid hydrogen
A full 160,000-cubic-metre cryogenic
liquid tank (a standard size on LNG vessels where liquefied
natural gas needs to be kept at minus 162°C) would contain 109,248
tonnes of liquid ammonia, which, using Shiowaza’s costings of
$0.48/kg, would cost $52.44m to produce.
The same tank would only be able to hold
11,376 tonnes of liquid hydrogen, which would cost $81.34m to
produce at a price of $7.15/kg.
The ammonia shipment would therefore
contain 596.8GWh of energy, at a cost of $87.87/MWh, whereas the
hydrogen tankful would contain 404.8GWh at a cost of $200.94/MWh.
Green Play Ammonia™, Yielder® NFuel Energy.
Spokane, Washington. 99212
www.exactrix.com
509 995 1879 cell, Pacific.
Nathan1@greenplayammonia.com
exactrix@exactrix.com
|